NEWS Climate Change is an Energy Problem. Here’s How We Solve It.
For the California Magazine article, click here.
Count on comedians to nail the zeitgeist.
I’m thinking of comics like Marc Maron, whose act riffs off existential pain points like mortality, antisemitism, the delaminating geopolitical situation, and, of course, that multigigaton carbon elephant in the room, climate change.
“The reason we’re not more upset about the world ending environmentally, I think, is that, you know, all of us in our hearts really know that we did everything we could,” Maron deadpans. “We brought our own bags to the supermarket,” he says, then pauses a few beats.
“Yeah, that’s about it.”
No surprise that comedians are able to play our eco-dread for yuks. Comedy is often rooted in the fertile manure of uncomfortable truths: we laugh so we don’t sob. And that’s all fine and good; laughter’s a good antidote to the malaise that comes from doomscrolling our newsfeeds day in, day out.
But are we really ready to throw in the towel and laugh ourselves into oblivion? And is Maron correct? Have we really done nothing to confront our foremost environmental crisis? Hardly. True, we haven’t yet reversed the upward trend in greenhouse gas emissions, and the challenge of transitioning away from fossil fuels often seems insurmountable. Is it, though?
According to Berkeley experts interviewed for this story, there’s reason for hope that we’ll make it through the bottleneck yet. The technology is already here and improving all the time. It won’t be easy, but it is doable. Now, let’s see how:
SOLAR
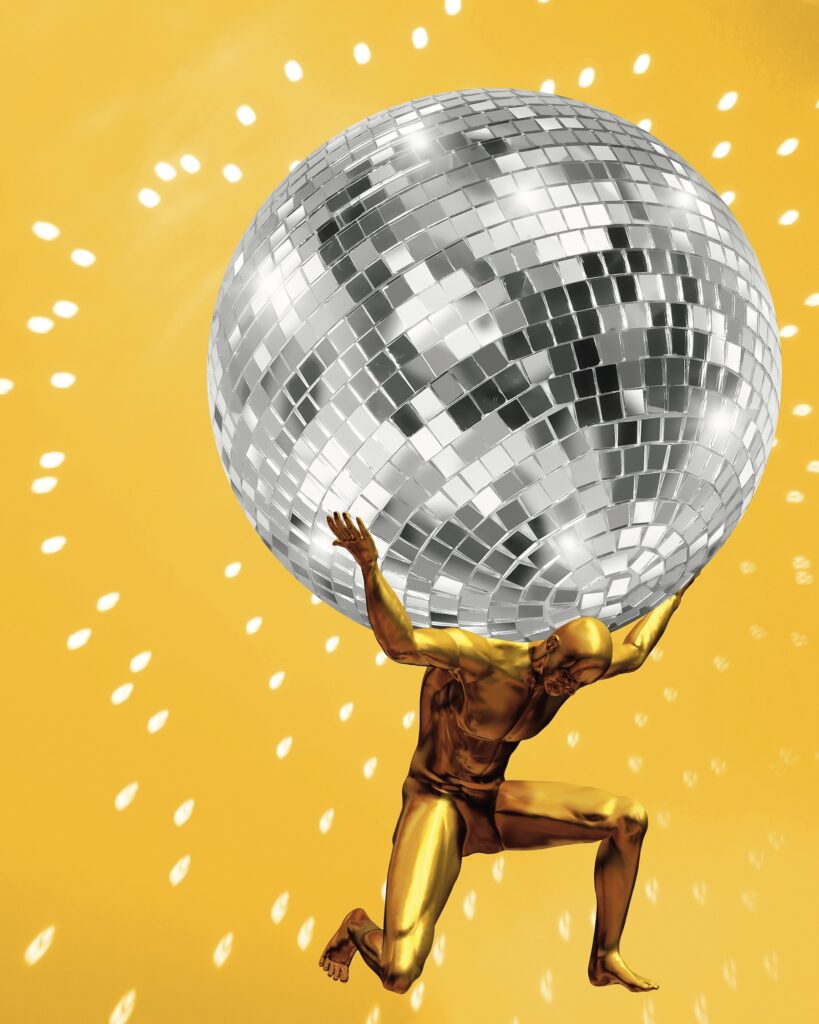
If you’re looking for a peg to hang your hopes on, start with energy economics and, in particular, the price of solar panels. Costs have dropped by nearly 90 percent since 2009, driven by both improved technology and global production (particularly from China). In 1976, solar electricity cost $106 a watt; today, it costs less than 50 cents per watt. Bottom line: Solar is now competitive with fossil fuels as a means of energy production.
While solar still only accounts for 3.4 percent of domestic energy consumption, production has been growing by more than 20 percent annually over the past five years, and likely would have been higher if not for shipping and supply chain difficulties stemming from the pandemic.
Production isn’t everything, however. For widespread adoption, an energy source must be available on demand. And it’s here that fossil fuels have a big leg up. Natural gas or coal can be burned at any time to generate electricity as required. Solar panels produce only when the sun shines. Storing adequate energy for later use—i.e., at night or on cloudy days—has long posed a major obstacle.
Solar production has been growing by more than 20 percent annually over the past five years, and likely would have been greater but for the pandemic.
Not anymore, says Daniel Kammen, the founding director of Cal’s Renewable and Appropriate Energy Laboratory and a professor in the Energy and Resources Group and the Goldman School of Public Policy. A coordinating lead author of the Intergovernmental Panel on Climate Change since 1999, he shared in the 2007 Nobel Peace Prize.
“I don’t see storage as a major problem at this point,” Kammen says. “It’s not a single breakthrough that makes me think that way, but more that we’re seeing the same trend in price and performance for storage that we saw with photovoltaics. A variety of approaches are coming to market, and they’re scaling really fast. Things that used to take several years to develop now take a year, and that’s almost certain to continue.”
The storage of the future will serve two different sectors, observes Kammen: transportation (think electric vehicles) and everything else (homes, office buildings, factories, etc.).
EVs
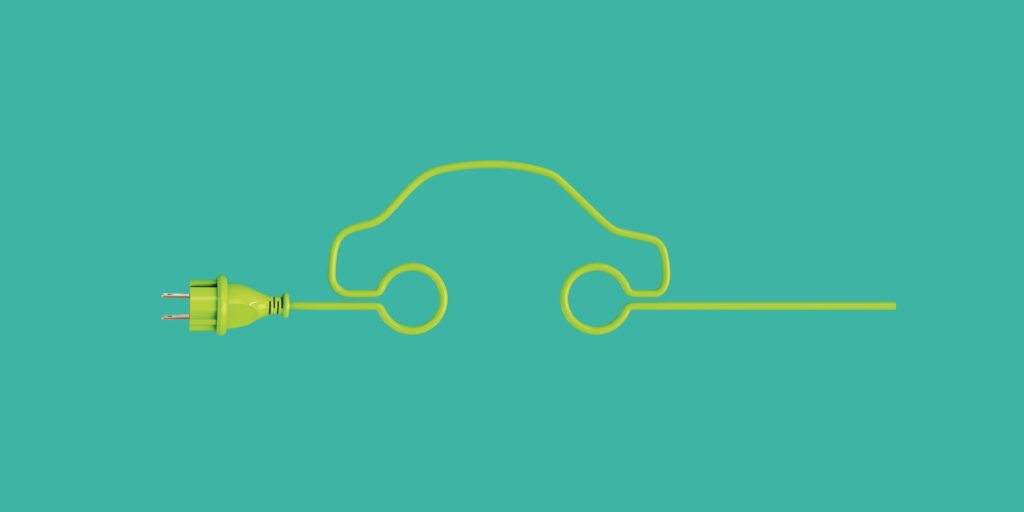
From a climate change point of view, an electrified vehicle fleet is desirable because it dovetails nicely with a green electric grid—i.e., one fed by sustainable energy sources. Currently, cars burning gasoline or diesel spew about 3 gigatons of carbon into the atmosphere each year—about 7 percent of total human-created CO² emissions. Just electrifying roughly a third of China’s vehicle fleet could slash carbon emissions by a gigaton a year by 2040. So there’s a lot at stake with electric vehicles, and everything considered, Kammen is pretty sanguine about their progress.
“It’s really been picking up, particularly over the last year,” he says. “It’s probably not a coincidence that gasoline and diesel prices have been spiking at the same time, and I hate to think that the war in Ukraine is part of that, but it probably is.” EVs are now the best-selling cars in California, Kammen continues, “and it’s the same in Norway, and it’ll soon be the same in New York. Prices on EVs are coming down. The trend is strong and accelerating.”
EVs generally store energy in batteries that use lithium, a relatively rare element that charges and discharges rapidly and is lightweight—an essential quality for automobiles, where excess weight is anathema. Lithium battery technology is well advanced, and some EVs can now go 400 miles between charging, alleviating earlier anxieties about limited range.
A central goal of the Biden administration is the construction of 500,000 new EV charging stations. For perspective: There are currently fewer than 150,000 gas stations in the entire United States.
The next challenge to overcome is a paucity of charging stations, a reality that still gives Tesla drivers pause before embarking on a long road trip. But that’s being remedied, Kammen says, thanks in significant part to the 2022 Inflation Reduction Act (see sidebar), which provides generous home and business tax credits for new and used EV purchases and fast-charging EV stations. A central goal of the Biden administration is the construction of 500,000 new EV charging stations distributed across all 50 states and the District of Columbia and Puerto Rico by 2030. For a little perspective on how ambitious that number is, consider: There are currently fewer than 150,000 gas stations in the entire United States.
“Worries over charging station access are real, there’s no denying it,” says Kammen. “But this legislation, coupled with the fact that recharge times are now very fast, will make a huge difference. The one thing that we still have to address, though, is the social justice component,” as not all zip codes will see the same resources. Without policies to ensure otherwise, Santa Monica will likely have charging stations aplenty; South Central Los Angeles not so much.
“We really need to ensure that doesn’t happen,” says Kammen. “First, it’s wrong. Second, to make a real difference, both energy production and transport must progress across a broad scale. That’s an easier case to make when everyone benefits.”
BATTERIES
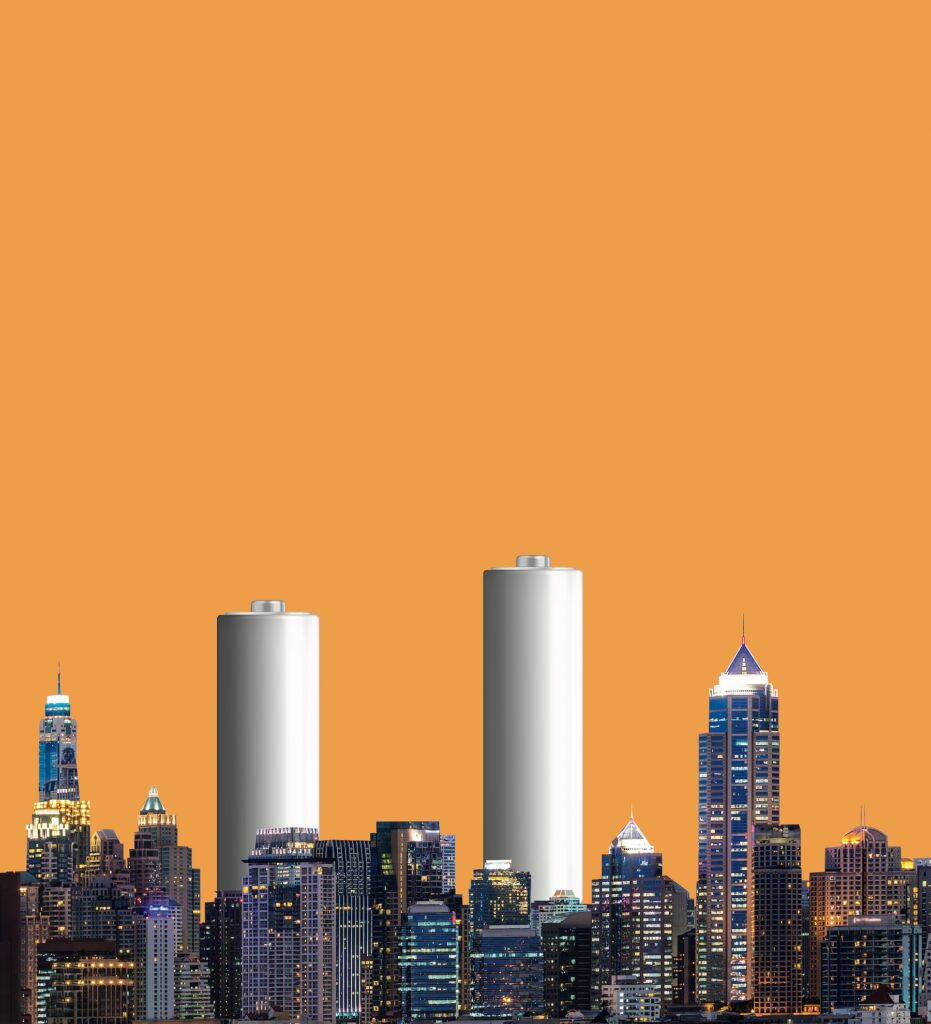
In addition to transportation, urban infrastructure must transition to sustainable, carbon-free energy as well. That will require combining clean energy with adequate storage to provide “grid reliability”—that is, systems that will keep the juice flowing in all seasons, even when the sun is absent or the wind stops blowing. In short, you need really, really big batteries.
But what kind of batteries? Lithium-ion batteries, already well established, are one option, says Kammen. But the qualities that make them ideal for vehicles—lightweight, fast charging capabilities—aren’t as critical when you’re trying to light a city at night. For stationary power needs, batteries can be industrial scale—heavy, with a large footprint.
Another problem with lithium is its scarcity. The United States currently controls less than 4 percent of global reserves. For that reason alone, researchers are looking for alternatives: batteries that employ cheaper and more readily available elements.
One of the most promising approaches, according to several sources, is iron-air batteries. And one of the leaders in the technology is Form Energy, a company headquartered in Massachusetts with satellite facilities in Berkeley.
Zac Judkins ’06 is the company’s vice president of engineering. He stresses that Form was obsessed with finding a way to address the problem of multiday storage, not enamored of a particular technology.
Judkins and colleagues evaluated a wide array of candidate chemistries before settling on iron-air batteries, which work by rusting and unrusting thousands of iron pellets with every cycle.
“When we started up in 2017, we saw that the world was rapidly moving to renewables—mainly solar and wind—and setting increasingly ambitious grid reliability and decarbonization goals.” Without effective storage, however, progress was going to hit a brick wall, Judkins says.
Analyzing the market, Form’s engineers arrived at a target. They needed to build a battery that could continuously discharge for 100 hours at a total cost of $20 per kilowatt-hour and had a round-trip efficiency (the amount of energy stored in a battery that can later be used) of 50 percent.
Those parameters, Judkins says, would allow for very high adoption of renewables with no sacrifice to grid reliability and minimal increase in cost to consumers. “That was the benchmark we had to hit.”
Judkins and colleagues evaluated a wide array of candidate chemistries before settling on iron-air batteries, which work by rusting and unrusting thousands of iron pellets with every cycle. Says Judkins, “We didn’t invent the iron-air battery. It was developed by Westinghouse and NASA in the late ’60s and ’70s. They’re not good for cars—they’re not light, and they don’t discharge rapidly. But there are advantages. For one thing, iron is abundant. It’s cheap. We don’t have to worry about supply constraints.”
What you also get with iron, says Judkins, is low cost and high energy density—i.e., the amount of juice you can put into the battery. The tradeoff is lower power density—how fast you can pull the energy out relative to volume.
“It’s roughly 10 times lower on power density than lithium-ion, but for our needs it’s fine,” says Judkins. “This is storage for large-scale, grid-tied projects.” Take the example of a large photovoltaic array like those on California’s Carrizo Plain. One array there has a 250-megawatt capacity, enough for about 100,000 homes, but only when the sun is shining. At night, during storms, there’s no electricity. But, says Judkins, with the addition of a Form plant with a footprint of 100 acres or so, you could store enough energy to keep the electricity flowing for a four-day period.
The company is now transitioning from proof of concept to full production. Ironically, the first commercial rust/unrust battery systems will likely come out of the Rust Belt. “We’re building a factory in West Virginia on a 55-acre site—a former steel plant—that will have approximately 800,000 square feet of production space and employ 750 people at full operation.” Green jobs. Once the plant is fully on its feet, Judkins says, it will produce 50 gigawatt-hours of storage capacity every year.
MICROGRID
In sub-Saharan Africa alone, 600 million people live without electricity. Providing them carbon-free power will require microgrids.
Large, centralized utility grids are naturally the focus for decarbonizing developed countries—but they don’t really apply to parts of the world where access to electricity is still rare. In sub-Saharan Africa alone, 600 million people live without electricity, which doesn’t mean they don’t want it. Providing carbon-free power to these communities will require microgrids: small systems that serve neighborhoods, hamlets, or even multiple villages. But while the microgrid concept has been kicking around for years, its full realization has been elusive—until recently.
“What we’re seeing is a meshing of enabling technologies,” says Duncan Callaway, an associate professor of Energy and Resources at Berkeley and a faculty scientist at Lawrence Berkeley National Laboratory.
For starters, he points to cheap solar. “With the profound price drop in panels, it’s a truly affordable resource that’s ideally suited for mid-latitude countries,” which experience less seasonality. “In general, you can serve electric demand with solar better in those latitudes than in countries [closer to either pole], where there’s just less sunlight.”
Another driver is cheaper, better storage options, Callaway says. For microgrid-scale, lithium-ion batteries work well. And these, too, have grown more affordable. “The explosive growth in electric vehicles really pushed things along,” Callaway says. “Ten years ago, it cost $1,000 for one kilowatt-hour of storage. Now it costs less than $100.”
Finally, says Callaway, “smart grid” technologies have been developed that make microgrids, once notoriously balky, highly efficient.
“We now have ‘big bucket’ control systems that allow for the smooth coordination of energy production, storage, and demand,” Callaway says. “That makes these small grids both low-cost and really reliable. The goal is to make systems that are truly modular, so you can plug various components into larger systems. That will allow easy customization and scaling.”
More than 150 microgrids already are deployed in the United States, powering everything from individual buildings in large cities to small, remote villages in Alaska.
As far as widespread adoption goes, Callaway doesn’t foresee many technical difficulties. It’s social and political roadblocks that need to be overcome. “The great thing about microgrids is that they work well in remote, underserved areas and they can be managed locally. But in less developed countries, there are often corrupt governments that want their cut from any project. And if that’s the case, you’d have an inherent bias toward centralized grids with baseline power plants.”
It’s a challenge that must be met, says Callaway. “Somehow, some way, small grid technology must be put on a level playing field with the old system, the large, centralized grid—or it’s unlikely to make it, even where it’s clearly the superior choice.”
FUSION
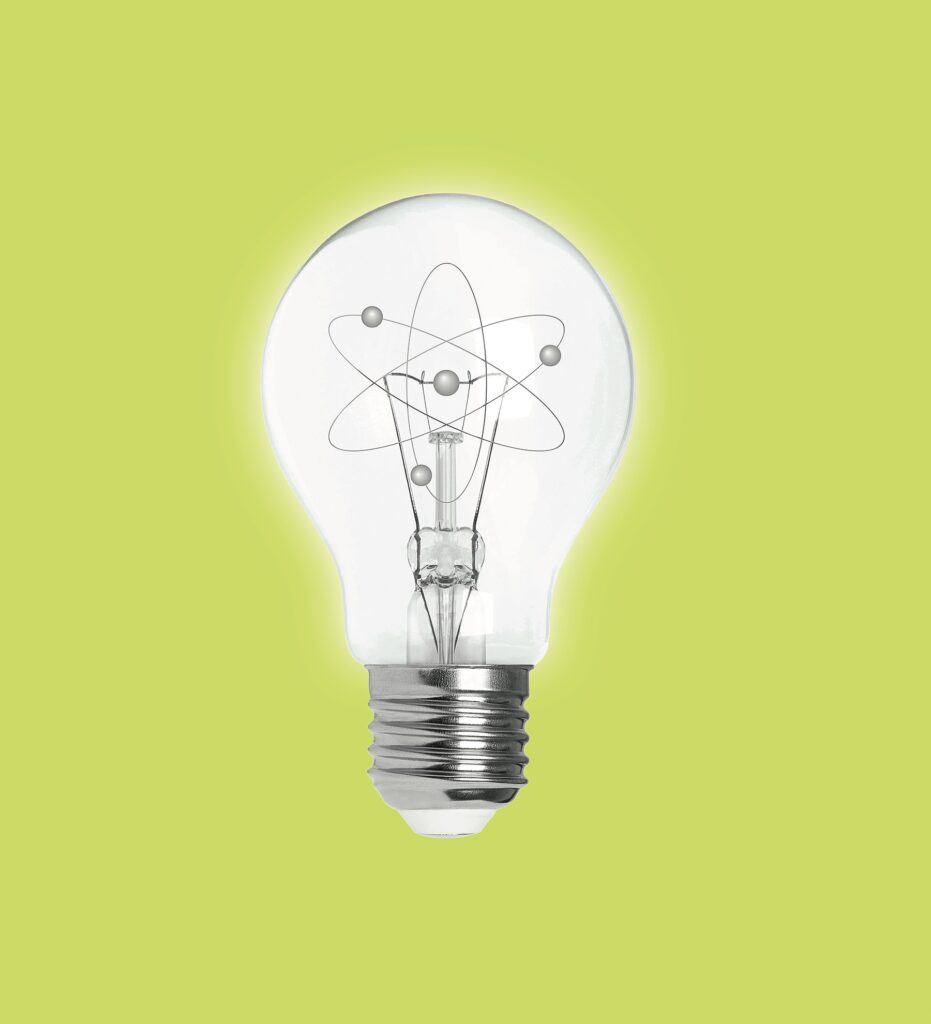
Microgrid or macrogrid, we’ll need a lot of clean, sustainable energy flowing through the wires if we’re going to simultaneously sustain an advanced civilization and cool the planet. Kammen is convinced it will largely come from fusion. But by that he means fusion in all its forms, including, as noted, the sun: that massive reactor in the sky that continually fuses hydrogen into heavier elements, releasing 3.8 x 10²6 joules of energy every second.
But there’s also that will‑o’-the-wisp that’s been tantalizing futurists and physicists for decades: terrestrial fusion reactors. These would use hydrogen—the most common element in the universe—as feedstock to generate gigawatt-hours of cheap energy, producing harmless, inert helium as the primary by-product. (Radioactive tritium would also be generated, but it has a short half-life and it’s consumed by the reactor in a closed-loop process.) Fusion technology remains the Holy Grail of clean, Earth-friendly energy production, but it’s also the butt of waggish comments. The most common is that it looks promising, but it’s 20 years away. And it’s been 20 years away for 60 years.
But after a breakthrough on December 5, 2022, at Lawrence Livermore National Laboratory’s National Ignition Facility (NIF), it now seems highly possible that a commercial fusion reactor actually could be available in, uh, well, 20 years. Maybe sooner.
Most fusion efforts to date have involved tokamak reactors—toroidal vacuum chambers that corral hydrogen atoms via magnetic coils, subjecting them to heat and pressure until they become plasma, a superheated (as in 150 million degrees Celsius) gas that allows the hydrogen to fuse. This releases energy that transfers as heat to the chamber walls, where it is harvested to produce steam to drive turbines for electricity production.
For the first time on this planet—other than during a thermonuclear explosion—a fusion reaction was created that produced more energy than was required to initiate the process.
Tokamaks have been able to coax hydrogen to fuse for brief periods—indeed, progress has been steady, if plodding, since the first machine was built 60 years ago. But to date, they haven’t been able to achieve “ignition”—that point at which sustained fusion occurs, and more energy is produced by the device than it consumes.
NIF took a different approach. Researchers there fabricated a minute pellet from frozen deuterium and tritium (both hydrogen isotopes). They then placed the pellet in a small gold capsule known as a hohlraum, which in turn was situated on an arm in a chamber bristling with 192 lasers. The scientists then fired the lasers simultaneously at the hohlraum, causing the inner capsule to compress. The result: temperatures and pressures exerted on the deuterium/tritium admixture were extreme enough to produce ignition. For the first time on this planet—other than during a thermonuclear explosion—a fusion reaction was created that produced more energy than was required to initiate the process.
True, the sustained yield was modest. The reaction lasted less than a billionth of a second and released 3.15 megajoules of energy, or slightly less than one kilowatt-hour. Not very much, in other words; the average American household uses about 900 times that every month. Still, it was 50 percent more energy than was expended by the laser bursts. Progress! But here’s another catch: While the actual laser beams represented only around two megajoules of energy, it took about 300 megajoules to power up and operate the mechanisms that fired the beams.
So, there’s still a lot to be done before we’re microwaving our frozen burritos with fusion power. Nevertheless, Kammen, ever the optimist, is fairly sure we will be soon.
“Given the trends, I think I’m pretty safe in predicting that we’ll derive about 70 percent of our power from fusion by 2070,” Kammen says. “Half of that will be from the sun and half from fusion power plants.”
And while NIF’s laser-blasted pellet approach points to future success, don’t rule out tokamaks. Kammen says he’s “expecting some exciting announcements about tokamak reactors pretty soon.” You heard it here first.
Solar fusion, too, will follow multiple avenues toward fuller implementation.
“It’s not just rooftop panels in cities and solar farms out on the landscape,” he says. “There’ll also be marine solar—large arrays out in the ocean.”
Also: orbital solar. Live trials are now underway at Caltech and the Jet Propulsion Laboratory, says Kammen, to establish large, autonomously assembled (i.e., no live astronauts required) solar arrays in space. The energy would be beamed down as microwaves to terrestrial collectors, where it would be converted to electricity. That may raise the specter of a loose-cannon death ray immolating cities from orbit if something goes awry—but not to worry, says Kammen. “The watt-per-square-meter dose is pretty low, so there’s no danger of anyone getting fried if they’re hit by it.”
He also thinks the fusion technology now under development for terrestrial reactors will have applications for space travel. “There’s a dual angle on fusion that’s really catapulting the technology,” Kammen says. “For better or worse, it’s imperative that we colonize the solar system so our fate as a species isn’t completely tied to one planet. Fusion propulsion will be an excellent means for getting us to the moon and Mars and beyond, and fusion—solar, reactor, or both—will also serve as a base-load power source when we get there.”
FISSION
Fission generates a lot of energy from a small footprint. Diablo Canyon, California’s sole nuclear plant, produces almost 10 percent of the total electricity consumed in the state, and it does it within a confine of 600 acres.
With all the fuss over fusion, the other “nuclear” power source, fission, seems to have faded into the background. That’s illusory. Fission is still quite hot, so to speak, with increasing numbers of erstwhile foes in the environmental community now embracing it—or, at least, tacitly supporting it. The reasons are clear. First, fission can generate a great amount of energy on a small footprint. Diablo Canyon, California’s sole operating commercial fission plant, produces almost 10 percent of the electricity consumed in the state and does it within a confine of 600 acres. And from a climate change perspective, nukes are peerless: they emit zero CO².
Of course, people remain worried about other kinds of emissions, such as intense radioactivity from long-lived waste isotopes. And older generation plants—that is, most of the ones operating today—are susceptible to core damage to varying degrees, with catastrophic results à la Chernobyl and Fukushima.
Those concerns are entrenched, especially in the United States, where environmental issues, regulatory red tape, and simple cost often conspire to scotch large infrastructure projects in the proposal phase.
“We’re pretty bad at megaprojects in this country,” says Rachel Slaybaugh, formerly an associate professor in nuclear engineering at Berkeley and now a partner at venture capital firm DCVC. “For one thing, it’s incredibly easy for them to go over budget. Just look at the new Bay Bridge, which ran triple the original estimates.”
That problem is compounded for nuclear plants, given heightened safety concerns and the regulations and litigation they engender. But there has been an upside to the impediments imposed on traditional nuclear power, Slaybaugh says: Out of necessity, more efficient—and perhaps more socially acceptable—technology has been developed.
The newer reactors are smaller—some much smaller—than the behemoths of yore, and pilot projects are underway.
“A good many of these designs originated from basic concepts developed in the 1950s or 1960s, but their refinement and commercial deployment is being driven in large part by our inability to construct large projects,” Slaybaugh says.
Different reactors have been designed for different situations, Slaybaugh observes, employing various fuels, coolants, and configurations. Some “breeder” reactors could even burn their own byproducts, greatly reducing radioactive waste.
“What’s the priority?” Slaybaugh asks rhetorically. “Economics? Providing high-temperature heat, or balancing renewables on the grid? Minimizing nuclear waste? A combination of different goals? These new designs can be standardized or customized and scaled for the site and requirements, and all involve considerable engineering to ensure safety.”
Some of the reactors will be large enough to power a city, or several cities. “And others will be teeny,” Slaybaugh says. “Those will be perfect for remote military bases or research facilities, say Antarctica or the Arctic. You’d eliminate several major problems with one of these very small reactors. Think of the logistical difficulties involved in getting diesel fuel to an arctic base, not to mention the heavy pollution it produces and, of course, the CO² that’s emitted.”
Fission technology also has some profound advantages over renewables, she says. “There are real limits to how many solar farms and wind turbines we should or even can build,” she observes. “A lot of materials are required for their production, and a lot of mining is needed to get the necessary elements. And these facilities tend to have very large footprints. I’m actually worried that we’re going to see a strong solar and wind backlash as people really start to understand all the impacts.”
Every energy source has strengths and weaknesses, continues Slaybaugh, “and we need to have sophisticated conversations on what they are and where each can best apply. Ultimately, my view of fission is that it’s a necessary tool that we must use in conjunction with other available tools to get the job done as well and as quickly as possible. No single solution is going to work for all scenarios.”
CARBON REMOVAL
At this point, we know what we must do to turn things around. Even better, we have the technologies and techniques to do it. But we need to deploy them.
Reducing carbon emissions is not the complete solution to global warming, say scientists. To really get a handle on the problem, we’ll also need to remove existing CO² from the atmosphere and sequester it permanently in the ground. One option, direct air capture (DAC), is the basis for a small but growing industry: Currently, there are about 20 DAC pilot plants operating, in total capturing and sequestering around .01 megaton of atmospheric CO² annually. According to the International Energy Agency, that storage could grow to 60 megatons a year by 2030, assuming large-scale demonstration plants proceed apace, current techniques are refined, and costs drop as the technology scales.
But those are a lot of assumptions for minimal benefit. Granted, a 60-megaton mass of anything is impressive. But from a climate-change perspective, 60 Mt is negligible, given energy-related carbon emissions hit an all-time high of over 36.8 billion tons in 2022. Many researchers think there are better options, and we don’t have to do anything to develop them because they already exist. They point to natural carbon sinks: forests, wetlands, grasslands, and, most significantly, the oceans. These natural systems are part of the Earth’s carbon cycle, which absorbs and releases about 100 gigatons of carbon a year. A planetary mechanism of that scale might seem more than adequate to handle carbon emissions, and it would, if atmospheric CO² only originated from natural emission points such as volcanoes and hydrothermal vents. As noted recently by MIT professor of geophysics Daniel Rothman, natural sources contribute ten times more carbon to the atmosphere than human activities, but it’s the anthropogenic carbon that is pushing the cycle over the edge. The planet can’t process the extra atmospheric carbon back into a stable earthbound state fast enough.
This deficit is exacerbated by the fact that we’re degrading our carbon sinks even as we’re pumping more CO2 into the sky.
“The ecological services carbon sinks provide are really priceless,” says John Harte, a professor of the Graduate School in Berkeley’s Energy and Resources Group. Harte, who conducted pioneering work on the “feedback” effect a warming climate exerts on natural carbon cycles in high-altitude meadows, observes that carbon sinks were poorly understood 35 years ago.
“But we now know they absorb 18 billion tons of CO2 a year. Realistically, we should be putting more of the money we’re devoting to the development of carbon sequestration technology into enhancing natural carbon sinks. At the very least, we need to stop their degradation.”
Harte’s work in the Colorado Rockies entailed artificially heating plots of land and tracking changes in vegetation types and carbon sequestration rates. In plots that weren’t heated and experienced climate change in real time, he found that wildflowers dominated, cycling large volumes of carbon into the soil during the short alpine growing season; when the plants died back each fall, the rate of carbon storage dropped off dramatically. But as Harte warmed specific plots over a period of years, woody shrubs replaced the flowering annual plants earlier than on nonheated land. These slower-growing plants sequestered carbon at a much slower rate than the wildflowers.
“The ‘money,’ the carbon, in the bank account shrinks,” says Harte. But after about 100 years, you begin to see dividends. “The carbon coming into the soil from woody plants is stored longer, so you eventually still have carbon in the soil.”
The goods news: This suggests natural sinks could be managed for optimal storage. But if emissions remain high, they’ll strain and ultimately overwhelm the sequestration capacity of the sinks, negating their value.
“If climate change continues, if we don’t cut back on emissions,” says Harte, “there’ll be no way to buffer the effects.”
And really, that’s the crux of the whole issue. At this point in the climate change crisis, we know what we must do to turn things around. Even better, we have the technologies and techniques to do it. But we need to deploy them. That means everything: solar in all its forms, from rooftop panels to orbital microwave arrays; wind turbine farms, both on land and at sea; fusion reactors; fission reactors; microgrids; massively distributed storage systems. And we must enhance, not debase, the natural systems that sequester carbon. We need to plant many more trees and manage working forests more sustainably, calculating carbon storage as a product equal to or exceeding board feet of lumber. And we need to protect the greatest carbon sink of them all: the ocean.
“I’m terribly worried about the trend toward seabed mining,” says Kammen. “It’s the least regulated of all the new frontiers, some very large companies are pushing it, and it’d be absolutely devastating. If we don’t stop activities like that and if we don’t use all the sustainable energy options that are available, we are risking extinction.”
That may not be a very optimistic note to end on, but then, optimism only gets us so far, doesn’t it? What we need now is grit and determination.
You must be logged in to post a comment.